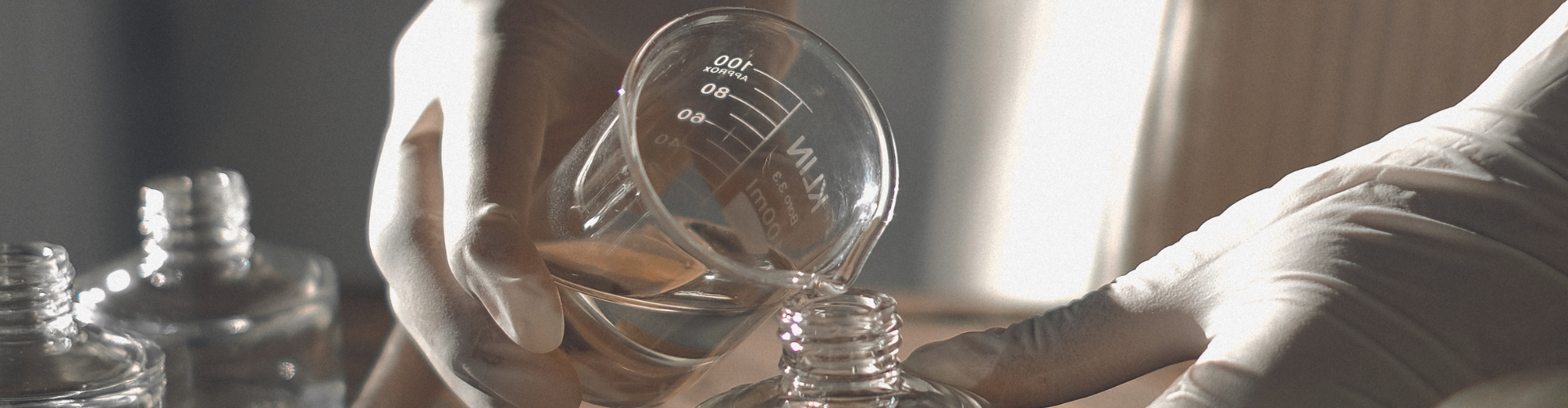
Sanitation and Treatment: A New Frontier for Health
Initially, the companies that brought running water into homes did not foresee the need to handle sanitation. It was only later that they had to take on this responsibility. Specifically, with the end of water scarcity—a period in which water resources were more about prestige and power than comfort and service—and the advent of the modern era, the volume of contaminated water multiplied significantly.
However, due to limited resources and political necessity, French cities were long reluctant to invest in sanitation. In 1909, only 10 percent of French cities with a population of over five thousand had implemented a comprehensive sewer system, compared to 36 percent of their German counterparts. Private companies stepped in to provide this service, contributing to a unified and fair development of France’s territory.
Grégory Quenet
British economist Angus Deaton, who was awarded the Nobel Prize in Economics in 2015, highlights sanitation as a key element in the “great escape” of humanity, alongside advances in nutrition and growth. By increasing life expectancy, “not only will almost all newborns survive to adulthood, but each young adult has more time to develop their talents, passions, and life, leading to a significant increase in skills and well-being.”1 This “great escape” is much more engaging than the one depicted in Jean Giono's novel, where a soldier seeks refuge on rooftops to escape cholera.2 The Saint-Simonian disciples of Prosper Enfantin could not have hoped for a more dazzling success.
However, they did not have all the keys to meet this challenge in 1853. The supply of water from outside the cities was not immediately accompanied by controlled wastewater disposal. Moreover, the development of networks initially neglected the treatment of water pollution, both upstream and downstream of its consumption. While ancient treatment techniques did exist, it took numerous innovations and significant financial resources to ensure the long-term health of populations and the increase in life expectancy. How did territories address the challenge of transforming unsanitary water into safe, drinkable water? How did scientists find ways to sanitize wastewater discharged into the natural environment? With industrial, agricultural, and domestic effluents, as well as the impact of climate change on water pollution, what progress has been made in terms of treatment? Let's take a closer look at these advancements, made possible by generations of chemists and biologists.
The Beginnings of Water Purification and Treatment
With over seventy quality criteria, both sanitary and environmental, tap water has become one of the most regulated food products in France. However, the concepts of “potability” and “sanitation” vary depending on the era and scientific knowledge. Originally, “potable” water was directly drawn from rivers or underground aquifers without any further intervention. However, several millennia before Christ, people realized that turbid or odorous water could be unpleasant. “The concept of water quality and treatment has been known for a long time. The Egyptians used aluminum salts, alum, for coagulation, which was used for water treatment,” explains Philippe Hartemann, professor of public health at the University of Nancy.
The Middle Ages marked a period of regression in terms of water treatment. No major innovations in water treatment would appear for nearly a thousand years. On the contrary, wastewater and waste were directly discarded into the streets, contaminating sources of drinking water through runoff and infiltration. According to the National Library of France, the first mention of a sewer that was not open-air in Paris dates to 1325. It was a gallery that passed under the City Hall and drained into the Seine. This dark period, which sometimes receives exaggerated discredit, contributed to shaping the unsanitary conditions of cities. However, it came to an end thanks to the development of science and a major discovery in 1670: the microscope. From that point on, research rapidly advanced, as the microscope allowed scientists to observe tiny particles in water. Throughout the eighteenth century, water filters made of sponge, wool, or charcoal became more common in households. During the same period, wealthy landowners used large burnt wood tanks to store water in better conditions.
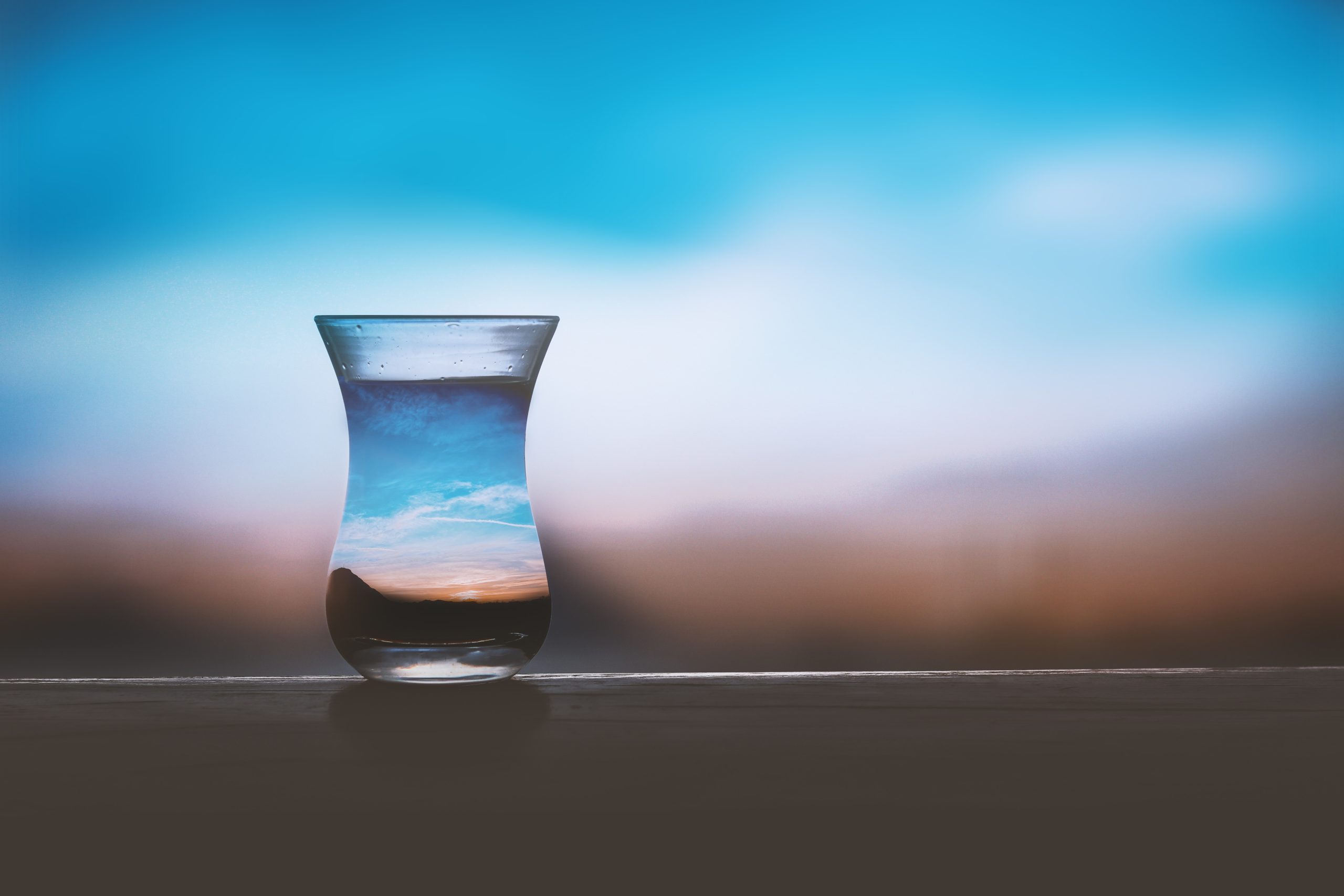
In the Mid-nineteenth Century, Circulation before Purification
It was through the building of circulating networks that the hygienic revolution first took place. The health movement, which began in the late eighteenth century, gained importance in 1832 during the first cholera epidemic in Paris. The severity of the disease, which killed thirty thousand people in Paris and one hundred thousand throughout the country, highlighted the importance of city sanitation. At that time, scientists adhered to the theory of miasma, which suggested that diseases were transmitted between individuals through a toxic vapor filled with particles. The first step was thus to create movement, and that's where the network came into play. As analyzed by Alain Corbin, according to their conceptions, “the model of the circulatory system, in an organic perspective, implies the imperative of air, water, and product movement. The opposite of ‘unsanitary’ is ‘movement.’ [...] The virtue of movement encourages channeling and the expulsion of filth. [...] Draining the city through sewers is disarming the putrid ancestral stagnation, preserving the future of the city, and ensuring, through technology, the regulation that nature alone cannot achieve in these artificially crowded places.”3
The discoveries made by British physician John Snow in London, although vigorously contested by the scientific community when he presented them, further emphasized the importance of a clean water supply. It was Snow who demonstrated the transmission of cholera through contaminated water during the 1854 London epidemic, rather than through polluted air. “He noticed that there were more sick people on one side of the street than the other. Through his epidemiological study, he demonstrated that the sick individuals had fetched water from a contaminated fountain. Once access to that fountain was prohibited, the epidemic disappeared,” recalls Philippe Hartemann. These discoveries encouraged cities to seek water sources other than their own wells.
In Paris, a strategy of diversifying water resources was being implemented in order to improve the quality of the drinking water. “Initially, we were seeking water from further or deeper sources to ensure its purity,” explains Séverine Dinghem, Director of Support for Professions and Performance at Veolia. To address the challenge of resource diversification, Baron Haussmann initiated the construction of aqueducts that would supply Paris with water from the sources of Le Havre or directly from the Dhuys. Water treatment was not considered the best way to obtain high-quality water. On the contrary: “At the time, Haussmann and his director of water services, Belgrand, made it a political objective to supply Paris with naturally pure and fresh water,” says Paul-Louis Girardot, former CEO and administrator of CGE. During the 1867 Universal Exposition, in the competition between Napoleon III and Queen Victoria, Haussmann and Belgrand demonstrated a renewed French superiority. To ensure this objective in the long term, Haussmann planned to extract water from the alluviums of the Loire and transport it to Paris by gravity.
In contrast, Bernard Barraqué, CNRS research director and water specialist, points out that the city of Lyon chose to draw water from its rivers or groundwater, emphasizing proximity. The Saint-Clair plant, operated by the Compagnie Générale des Eaux for the city of Lyon, treated water from the Rhône by naturally filtering it into two large underground basins. The management of wastewater also became a major concern in the French capital, where “the quality and collection of wastewater quickly became an issue to prevent contamination of drinking water distribution points,” says Dinghem. Moreover, as water consumption increased, the quantity of contaminated water also increased. To address public health needs, the first sanitation networks emerged in Paris during Haussmann's major projects, thanks to the ingenious sewage system devised by Eugène Belgrand.
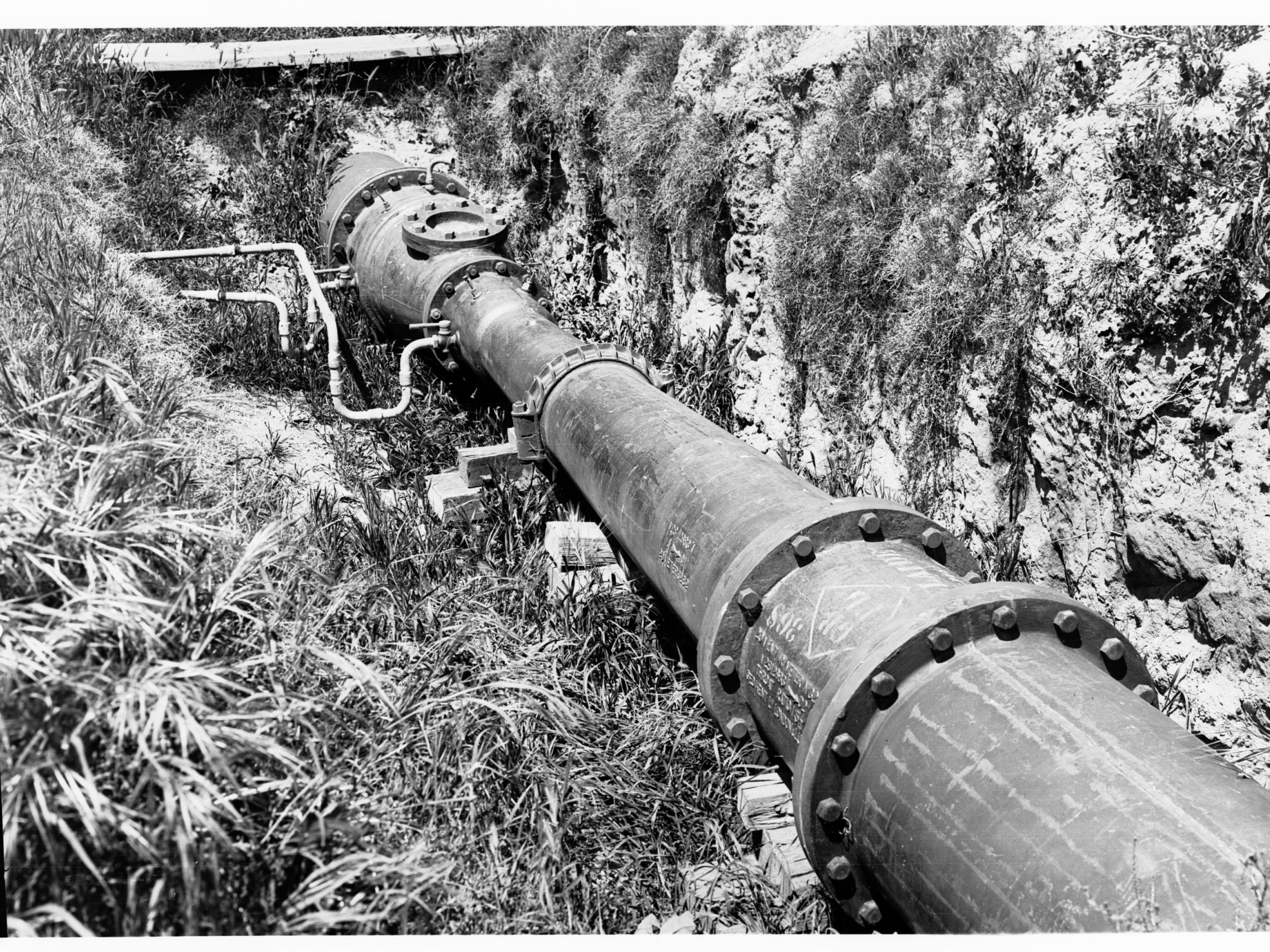
Haussmann, an engineer from the Ponts et Chaussées, transformed Paris by creating a unified double underground network: one supplied drinking water while the other eliminated wastewater through the sewers. Belgrand's objective for the sewer system was multifaceted: to evacuate rainwater, industrial waste, and household wastewater, and to enable cleaning of the galleries using valve wagons. Under each Parisian street, he coordinated the installation of a sewer. In total, the engineer constructed six hundred kilometers of sewers beneath the feet of Parisians. “What makes the Parisian network unique is its visitability. Thanks to this, even today, we can visually diagnose and locate a leak in the majority of the network at a low cost by conducting an inspection or even sending a drone,” says Dinghem.
In a less monumental manner, the Compagnie générale des eaux also developed its expertise and signed its first contract with Boulogne-sur-Mer in 1880, incorporating the management of wastewater. “Indeed, it planned for the construction of a sewer network, taking charge of the evacuation of wastewater and fecal matter that, until then, were discharged [...] into the front port and the grounding port,” historian Patrick de Gmeline writes4. It constructed sixteen kilometers of sewers to complement the city's infrastructure. The modern sanitation network that we know today naturally took time to emerge, but it took a major step forward from 1894 with the law that made sewerage mandatory.
Between Public Health Crises and Scientific Discoveries: The Beginnings of Modern Treatment
Initially, water networks were intended to fetch clear water in large quantities from outside cities, while sanitation networks aimed to dispose of putrefying water far away. In line with miasma theories, they merely created movement to prevent stagnation, ultimately expanding the circle of pollution identified by John Snow. At its inception, “the sanitary movement had no science to guide its efforts,” emphasizes Deaton5.
However, a scientific revolution emerged at the end of the nineteenth century, accelerating awareness of the need to treat the resource. Gradually, the miasma theory gave way to the microbial theory. The epidemiological work of the British John Snow was succeeded by the Germans Robert Koch and Karl Joseph Eberth, who laid the foundations of microbiology, and then by the Frenchman Louis Pasteur, who became one of its most famous figures.
Thanks to them, we can now say that “not everything that stinks is deadly, and not everything that is deadly stinks,” says Corbin6. The correlation between disease and water contaminated with microbes was scientifically demonstrated. “We drink 90 percent of our illnesses,” Pasteur declared in 1881.
These discoveries helped to understand the lingering health problems associated with the creation of networks and to provide solutions through the implementation of treatment methods. “Science eventually caught up with practice, and the microbial theory of diseases was gradually put into application through more targeted measures based on scientific grounds.” The history of modern treatment begins after the history of networks. However, as Deaton observes, “transitioning from the microbial theory to safe sanitation and water takes time and requires money and authority.” It also requires “engineering and monitoring skills to ensure that the water is truly uncontaminated.”7
Louis Pasteur, Father of Microbiology and Pioneer of the Hygienic Movement
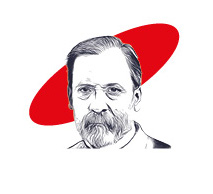
Louis Pasteur has left an indelible legacy in various fields of research, including public health and the role of water in hygiene. The chemist and physicist by training contributed to the collective awareness that water could contain microbes and transmit diseases.
In the collective imagination, Louis Pasteur is known for his intelligent eyes, gazing intently into the camera lens of Félix Nadar. He is also hailed as the father of modern medicine, the inventor of the rabies vaccine, and the namesake of the pasteurization process that extends the shelf life of our food and beverages. However, how many are aware of his pivotal role in ensuring the safety of the water we consume?
Born on December 27, 1822, in Dole, in the Jura region of France, Pasteur was admitted to the École normale (a French elite school) at the age of twenty-one, where he studied physics and physical chemistry. In 1847, he defended his doctoral dissertation in science at the Faculty of Sciences in Paris. Ten years later, he was appointed as the administrator in charge of studies at the École normale supérieure.
From biology to agriculture and medicine to hygiene, Pasteur distinguished himself in numerous fields and pushed the boundaries of scientific knowledge of his time, laying the foundations for our current understanding of germs and their role in disease. He dedicated a significant part of his career to studying waterborne illnesses caused by contaminated water and lack of sanitation, notably cholera.
Pasteur is remembered for his demonstration of the existence of microbes, which develop, among other places, in aquatic environments. After memorable battles against his opponents, particularly Félix Pouchet, a renowned biologist and staunch defender of spontaneous generation, Louis Pasteur published his work refuting this theory in 1861 and 1862. According to him, microorganisms present in atmospheric dust develop and multiply; no living being emerges from nothingness. Furthermore, these microbes can cause diseases and contaminate entire populations. Hence, they should be avoided and combated.
In doing so, Louis Pasteur contributed to the hygienic movement by emphasizing the importance of cleanliness, especially hand hygiene, and the role that water supply plays in combating diseases. Providing the evidence needed for the triumph of the microbial theory over the miasma theory, he also stated that water can carry diseases without being visible or detectable, and may require treatment to eliminate them.
By closely studying the life of microorganisms, he also laid the groundwork for early treatments, highlighting the role that filters or microorganisms themselves can play in self-consumption or annihilation—as seen in the principle of activated sludge, particularly used in wastewater treatment plants.
Pasteur's discoveries, like his predecessors', were not the result of spontaneous generation of ideas in the fertile mind of a genius; they were the fruit of experimentation, not without errors, and built upon a long series of scientific progress.
Pasteur's breakthrough in developing the rabies vaccine in 1885 brought him worldwide acclaim, and he received numerous honors. The Academy of Sciences proposed the creation of an institution dedicated to treating rabies, leading to the establishment of the Pasteur Institute in 1888. Pasteur passed away on September 28 in Villeneuve-l'Étang, in an annex of the Institute that bears his name. He left behind a profound transformation in our relationship with water, summed up in the apocryphal quote, “We drink 90 percent of our illnesses.”
Treating the Water We Drink: From New Techniques to Strengthened Surveillance
To ensure that the water reaching the taps of the French people is of the highest possible quality, drinking water treatment plants, particularly those operated by engineers from the Compagnie Générale des Eaux, initially equipped themselves with slow sand filtration systems. These systems first removed the turbidity of the water, which gives it its color. They were gradually complemented by sedimentation and coagulation devices, which settle particles and make them sink to the bottom of basins before the water passes through filters, significantly improving the quality of the distributed water. In Germany, Koch installed large sand filters to supply Hamburg with water, thus putting an end to a cholera epidemic. Thanks to the work of Louis Pasteur in the late nineteenth century, filters were able to better eliminate microbes. This applies to the Pasteur filter, named for Louis Pasteur but conceived by French biologist and physicist Chamberland. Equipped with a porcelain cylinder, it filters liquids and can retain the microorganisms in the water.
Engineers and leaders of the Compagnie Générale des Eaux are attentive to the numerous scientific developments and support initiatives aimed at increasing water treatment capacity in France. “After studying the filtration methods used in Germany and England, they concluded that they were not sufficient to obtain high-quality drinking water. They favored the coagulation method, known as the 'Anderson' process, based on iron,” narrates Patrick Gmeline8.
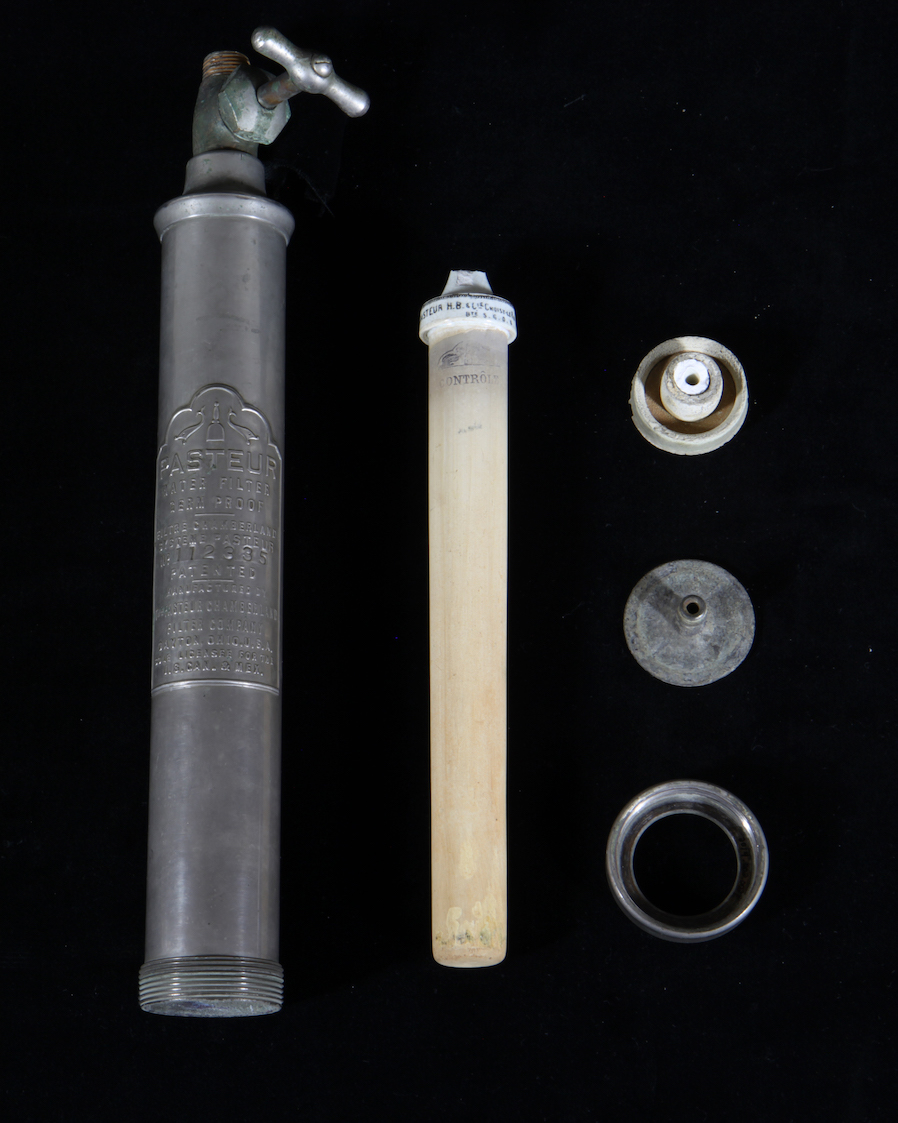
Dedicated water treatment plants multiplied, further transforming our relationship with water. It changed from being a natural resource within reach to a resource that is transported across distances, increasingly resembling a good that requires human intervention and transformation. In the capital, where water quality remained unstable, “the Compagnie Générale des Eaux was called upon to contribute to the improvement of the purity of Parisian water.” The Choisy-le-Roi plant, built in 1861, had slow sand filters from the 1890s onwards, a first of its kind in France.
Extensive work was “undertaken by the Company in the Méry-sur-Oise and Neuilly-sur-Marne stations,” and the first experiments on new filtration systems were conducted at the Boulogne-sur-Seine plant.
However, this was not enough. “In 1892, Paris and its suburbs were once again severely affected by cholera, which killed 1,800 people. Upstream of the capital, where the river water was relatively pure, cholera caused minimal casualties. Downstream of the sewer outlets, the mortality rate was much higher.” Two consequences followed. The first involved revising the general distribution scheme, which stipulated that the Company “consolidate its filtering plants upstream of the Seine” in Choisy-le-Roi. The second focused directly on the quality of the water produced, “drawn from areas known to be clean” and especially “purified by iron treatment followed by sand filtration.”9. In the early 1900s, physico-chemical treatments of water using ozone, ultraviolet light, or chlorine complemented the water filtration process with disinfection. These discoveries coincided with the Public Health Act of 1902, which, for the first time, obliged municipalities to comply with a number of water quality criteria. “Hygienists included the search for indicators of fecal contamination in the regulations. When they were found in the water, it was classified as a risk for human consumption,”10 explains Professor Philippe Hartemann.
Nice played a unique role in developing the first of these new treatments: ozone. Both in Nice and Paris, the quality of water delivered through the networks was unsatisfactory. A local chemist, Marius-Paul Otto, relied on the Dutchman Martin Van Marum’s discovery in 1781 of how to artificially create this gas made up of three oxygen molecules, and on its bactericidal properties, discovered by the German Ohlmüller in 1891, to improve water treatment. “The principle was simple: by producing ozone through electricity, one could greatly reduce the number of microbes and organic matter in the water... However, to make the process usable on a large scale, industrial production of ozone would be necessary,” Gmeline writes.11 And Otto achieved this.
Although the Compagnie Générale des Eaux was interested in Otto’s work, it initially remained cautious about this innovation and refused to commit to it financially—unlike what would happen later when they integrated the Compagnie Générale de l'Ozone, created by Otto, into their organization. However, progress was made on the operational front. The municipal council of Nice gave the Compagnie Générale de l'Ozone “the green light to implement its ozone process” at the Bon Voyage site, operated by CGE, in 1905. The ozone unit, the first of its kind, was put into operation in 1907. Two more units followed immediately in Nice and throughout the region. In just a few years, it was “considered everywhere as having the healthiest water in France.”12 The process would later be replicated throughout the country and abroad.
Beyond technical treatments, the importance of surveillance was emphasized by the typhoid fever outbreak that occurred in Lyon in 1928. The toll was heavy, with over three hundred deaths. “The cause was an aqueduct built by PLM, located between two collection wells, which over the years became a sewer into which the residual water from numerous villas in Vassieux, near the company's factory of the same name, was discharged.” Measures were taken, such as enhanced protection of the collection areas. However, to strengthen the quality of surveillance, “the establishment of a laboratory dedicated to the bacteriological quality of water” was implemented.13
Water Treatment of Discharged Water: From Spreading to Wastewater Treatment Facilities
The link between improving the quality of consumed water and that of the water being discharged was quickly established. In Paris, where Eugène Belgrand designed the system, wastewater was initially evacuated to two sites located in Asnières and Clichy, in the near suburbs of Paris. However, the 400,000 cubic meters of polluted water discharged into the Seine River each day created significant pollution. Eugène Belgrand then opted for an alternative solution: spreading. This idea was inspired by existing practices, as septic tank emptiers often resold the product of their purges as fertilizer.
“We realized that discharging wastewater into watercourses was not the right option, because even though they have a self-purification capacity, they only eliminate contaminants when they are present in a certain quantity. Discharging wastewater outside the cities was not sufficient; it needed to be treated when there was too much of it. In Paris, this began with the discharge of wastewater into fields,” explains Sophie Besnault, research engineer at the National Research Institute for Agriculture, Food, and the Environment (INRAE). The attempt was made to infiltrate wastewater into the soil of the Gennevilliers plain. “This was a preliminary treatment stage because we reused the nutrients in the soil to grow plants. However, we realized that there was not enough land to discharge all the wastewater; it became saturated. That's when we started building the first wastewater treatment plants,” adds Besnault.
The SARP, or the Transition from Evacuation to treatment
In 1937, Charles Dubreuil created the SARP: the Sanitation Company of the Paris Region. It was a vacuum truck company aggregated from smaller companies. Its goal was to benefit from the market share premium that existed in a sector that operated through word of mouth—the more clients there were, the more prescribers there were, and, in turn, the more clients there were—and to increase the quantities of excrement used as fertilizer for spreading. At that time, vacuum truck companies were created by farmers in search of fertilizer for their fields, such as CIG, which joined SARP in the 1980s: it was originally founded by a tulip farmer from Gonesse in the Val-d'Oise.
The success of SARP, which emerged in a fragmented sector of small players, symbolizes the change of an era, from a rudimentary approach to a more technical approach and from solely evacuating sludge to treating it. Trucks replaced men and horses; they were expensive and required funds.
Treatment centers, especially, with an expanding range of treatment options, needed volumes to operate. SARP sensed the changing times, which placed increasing importance on science. In the 1960s, it changed the meaning of its acronym to become the Rational Sanitation and Pumping Company. Driven by an entrepreneurial spirit, SARP then developed its activities by progressively penetrating a broad range of markets and by making itself a permanent field of innovation.
“Starting from individual septic tanks, we thought it would be beneficial to clean collective sanitation networks and offer solutions for emptying and cleaning industrial equipment,” recounts Marc-Olivier Houel, who was the CEO of SARP from 2013 to 2023. “And since the waste resulting from pumping was sandy, greasy, oily, and hazardous, we quickly implemented appropriate solutions to protect the environment, support our clients, and enhance our professional skills.”
On the vacuuming front, alongside the activity of cleaning individual septic tanks, which continued to thrive—France still has too low a population density to connect every household to the network, unlike many other Northern European countries, and still has at least four million septic tanks—the activity of cleaning city and industrial sanitation networks developed. In the 1980s, the first remote inspection techniques appeared, initially using VHS cameras “before resorting to drones today,” says Yannick Ratte, CEO of SARP in 2023. Other innovations appeared, such as the Vertigo technology which, by projecting an epoxy film inside the pipes, enables their rehabilitation without having to destroy the columns, to the great satisfaction of landlords.
On the treatment front, techniques were borrowed from the centers for hazardous waste treatment.
Such wastes accumulated with the development of industry: “Who else could Renault, foreseeing future environmental issues, turn to at the time to pump the residue from its painting tanks at its Boulogne-Billancourt factory? SARP,” Marc-Olivier Houel recalls. This allowed the Compagnie Générale des Eaux, which integrated SARP in 1970, to have initial solutions in place when it was confronted a few years later with pollution at the drinking water plant in Méry-sur-Oise, allowing it to treat them at the source and quickly giving birth to SARP Industries, which has since become a reference point in the treatment of hazardous waste.
At the same time, SARP launched the first treatment centers for dredging sludge, washing the sands and valorizing the greasy residues—a first in France. These developments and innovations position this Veolia entity at the crossroads of all its businesses today.
A major innovation of the early twentieth century was the discovery in 1914 by two British researchers, Edward Ardern and William Lockett, of free cultures or activated sludge. The principle: use naturally occurring bacteria to purify wastewater. “In basins, we inject air to sustain the bacteria. As they thrive, the bacteria consume nutrients. Through sedimentation, we separate these bacteria from the water, transfer them to large basins, and let them settle at the bottom,” explains Besnault. Even today, activated sludge, a natural solution, is the predominant process used for wastewater treatment in our country. “Half of the wastewater treatment plants in France operate with activated sludge. This is the case for all plants serving populations of more than ten thousand,” Besnault adds.
The first French wastewater treatment plant was established in 1940 in Achères, Yvelines, after three years of construction. In its early stages, the sanitation service was entirely dependent on the state. As Séverine Dinghem points out, “The private sector, including the Compagnie générale des eaux, was only involved in the collection and treatment of wastewater at a later stage because this public service was directly funded by the public, not by the users,” and the importance of the relationship with users in engaging private companies was recognized. Only later, after World War II and especially after the French Water Law of 1964, the invoicing of sanitation services to users, the increasing complexity of industrial pollution, and the development of technologies motivated the launch of public-private partnerships and the establishment of wastewater treatment plants across the country.
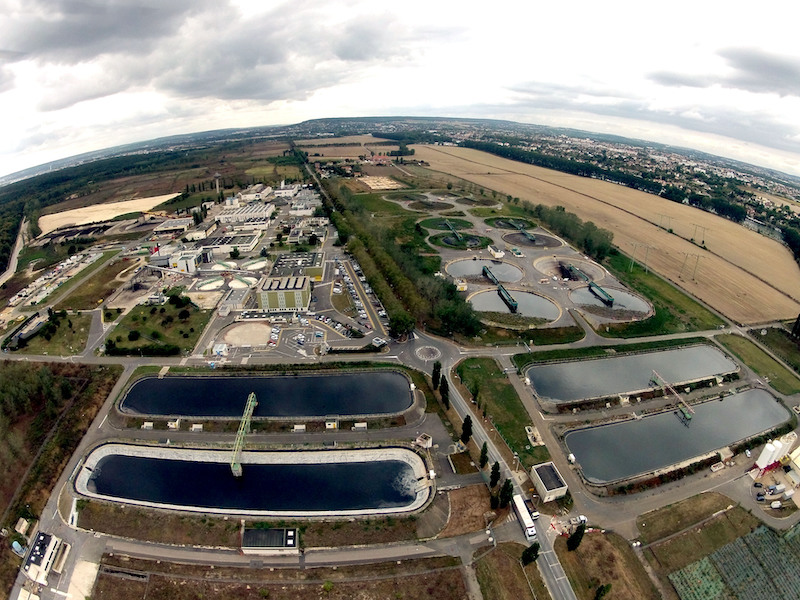
Water treatment plant in Achères, France.
© ToucanWings
Methods with similar foundations are used for the treatment of drinking water and wastewater: physico-chemical, biological, and chemical processes that rely on the oxidation of compounds. Since the early twentieth century, these methods have continuously progressed to consume less energy, occupy less space, treat larger quantities of water, and remove increasingly specific pollutants. Over the years, moreover, as pollution became more complex, these processes were combined to overcome the most stubborn problems, culminating in the 1970s in the production of drinking water directly from wastewater.
Rainwater: From Evacuation to Recovery
During the initial period of sewer system construction, the decision was made to collect rainwater and wastewater together for rapid evacuation from cities, aiming to reduce both diseases and flooding. This original choice of evacuation still underlies the most common sanitation scheme today.
However, this approach was not without disadvantages. During the sanitation process, it has become apparent over time that rainwater, polluted by runoff that carries pollutants as it washes over soils and buildings, may not actually be polluted enough for the optimal operation of wastewater treatment plants. “The problem is that for a wastewater treatment plant to function properly, it needs pollution,’’ says Cyril Gachelin, a specialist in rainwater and responsible for training at OiEau.
“With rainwater, the purifying bacteria are less effective in diluted environments, and the facilities are subjected to hydraulic overloads. We realized that rainwater caused certain dysfunctions in wastewater treatment plants.”
Since the 1990s, this approach has been refined: increasingly, rainwater and wastewater tend to be collected through separate networks. Rainwater is now considered “clear parasite water” for the sewer system. This means that rainwater poses risks of wear or overload to the pipes, excessive electricity consumption, and a decrease in the efficiency of wastewater treatment plants.
This evolution entails significant operational consequences and its impact must be assessed in each locality—separation requires extensive work on the water network, which is why this choice is rarely adopted by authorities, and wastewater treatment plants can be adapted in its absence.
In order to combat flooding, the infiltration of water into the soil has emerged as an essential complement to simply evacuating rainwater. In mainland France and the overseas departments, between the 1980s and 2020s, “between 200 and 250 square kilometers [of land were] impermeabilized annually, which is equivalent to a French department every twenty-five to thirty years,” according to the Ministry of Ecological Transition. However, soil impermeability, coupled with the more frequent occurrence of heavy rainfall, contributes to dangerously worsening the risk of flooding. Therefore, promoting the infiltration of rainwater directly into the soil is necessary.
Considering that 75 to 85 percent of the pollution in rainwater is due to runoff, this approach also helps limit pollution at the source and even harnesses the purifying power of the soil. “The current objective is to limit urban sprawl to reduce the phenomenon of impermeability. To achieve this, we need to make supermarket or cinema parking lots less impermeable. We need to restore infiltration capacity to rainwater, especially through vegetation,” explains Gachelin. In housing, the development of green roofs also increases retention areas where water can evaporate.
Finally, the question of rainwater harvesting arises. Knowing that only one percent of the water available on the planet is usable for human consumption, the billions of cubic meters of rainwater that fall are a resource that must be taken into account (though without depriving the soil).
“In France, we have regulations for indoor use,” Gachelin emphasizes. Rainwater can be collected within a dwelling, but it is not without constraints. It can be used to fill toilet cisterns, clean floors, and for laundry washing “provided that an appropriate water treatment system is used, a ‘non-potable water’ label is placed on the toilets, and it is reported to the local municipality, as some of this rainwater will eventually end up in wastewater.” It is not permitted for human consumption. Even for the authorized uses, “to be self-sufficient in rainwater, a very large storage capacity is required. However, it doesn’t rain every day, and the investments required for self-sufficiency are significant. Moreover, rainwater can be used for individual irrigation purposes outdoors.”
For professionals, the use of rainwater is allowed if the applications do not require potable water. In the summer of 2022, in France "car wash stations had to close due to water restrictions", Gachelin said. "For them, it can be beneficial to store large quantities of water or opt for closed-loop solutions to cope with these periods of drought.”
Individual solutions should be complemented with collective solutions, tailored to the needs identified by the local area. For example, Veolia initiated the storage of 300,000 liters of rainwater as a massive “cushion” (pond) in Nantes in 2012, to supply eleven street sweepers responsible for cleaning the city streets.
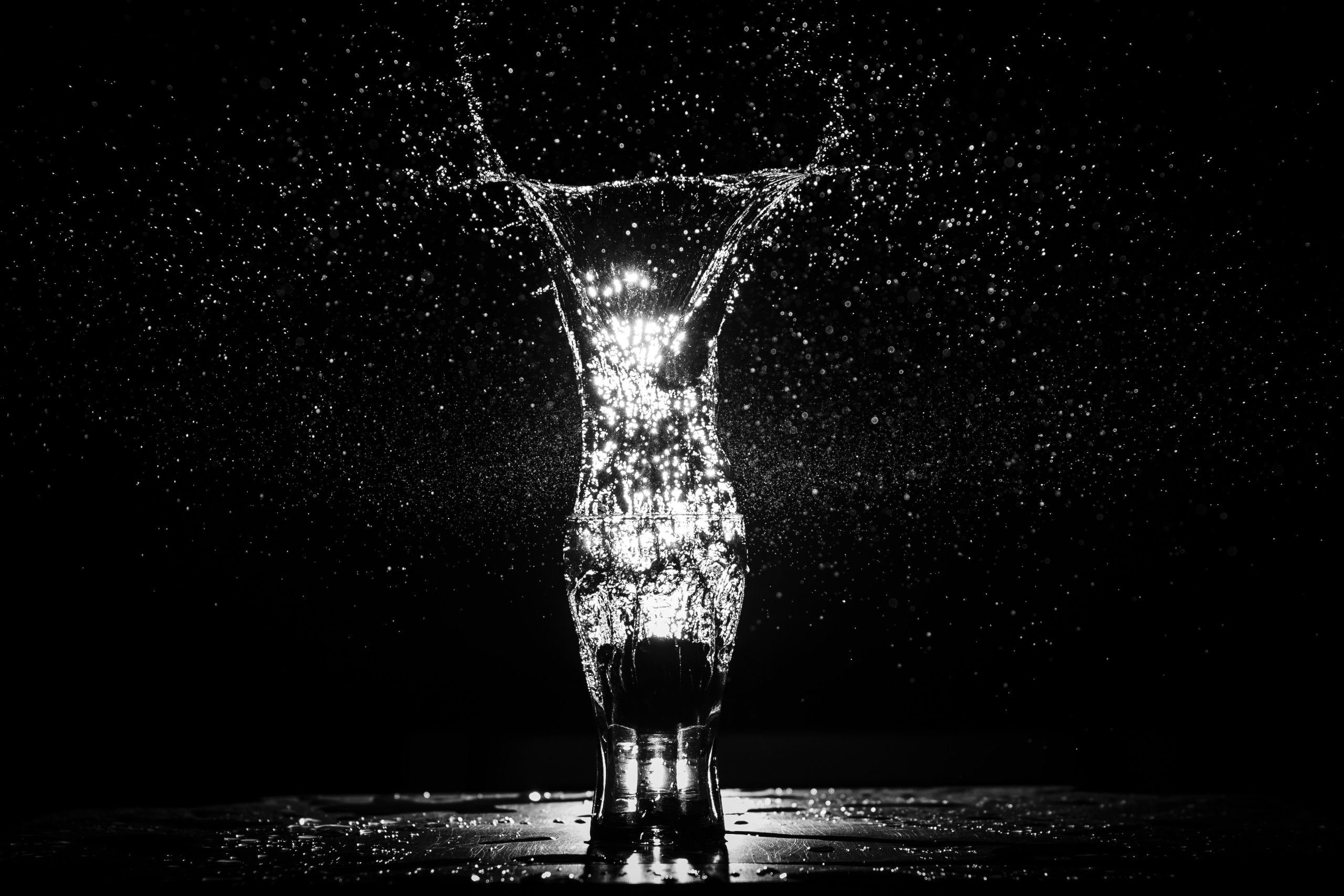
New Pollutions and Planetary Limits: Challenges for the Future
Today, water treatment strategies must confront multiple challenges. And, as has been the case for the past 170 years, health-related challenges persist. As pollution becomes increasingly complex, whether of agricultural, industrial, or even medical origin with the presence of pharmaceutical residues, the detection capabilities of particles are also improving. This opens up new possibilities for treatment.
Health: New Solutions in Detection and Prevention
Veolia actively participates in hazardous substance detection efforts. Since the adoption of a European directive in 2000, the search for hazardous substances in water discharges has been mandatory in wastewater treatment plants serving populations of more than ten thousand. To meet this requirement, the company, in partnership with Watchfrog, has developed a solution to detect potential toxicity related to the presence of endocrine disruptors or micropollutants in wastewater effluents. “To treat these micropollutants, we mainly use water treatment technologies at the outlet of wastewater treatment plants,” says engineer Sophie Besnault. “However, the treatment of these micropollutants requires a significant amount of energy and resources.”
Since the 1990s, water treatment plants and certain wastewater treatment facilities have implemented membrane ultrafiltration, which has revolutionized the field and continues to improve. Veolia is currently working on two types of filters with exceptional potential: carbon nanotubes and membranes that imitate fish gills. The aim is to make these technologies more accessible and energy-efficient. Overall, technological advancements now allow the filtration of pollutants that were undetectable just a few years ago. In Aarhus, Denmark, Veolia conducted an initial experiment in 2014 to treat pharmaceutical residues from a hospital and a municipal wastewater treatment plant. Using Moving Bed Biofilm Reactor (MBBR) technology, which utilizes microorganisms to degrade organic matter, 90 percent of the pharmaceutical residues were eliminated. The tests also demonstrated the need to prioritize the treatment of municipal wastewater, since people consume medications at home. But it is crucial to address these residues at the source: the best pollution is the one that is not produced.
“Beyond the numerous technical solutions available to identify, measure, and eliminate micropollutants in water, we must also consider changing mindsets,” explains Géraud Gamby, Water Market Director at Veolia. “By organizing awareness campaigns for citizens and economic actors and leveraging community networks, we need to focus on changing habits and practices.”
Environment: Contributing to the Respect of Planetary Boundaries
Water treatment processes, like all human activities, must adapt to climate change, which poses significant challenges in terms of water quantity and quality. “During drought episodes, water quality deteriorates with algal growth and increased concentration of matter. This makes water more challenging to treat, requiring stronger treatment steps,” says Hervé Paillard, Director of Process and Industrialization at Veolia.
Additionally, these processes must contribute to collective efforts to respect planetary boundaries, whether related to climate change, the freshwater cycle, nitrogen and phosphorus cycles, or biodiversity.
Research efforts to make water treatment processes more energy-efficient, less water-intensive, and better equipped to address environmental pollution play a role in meeting these challenges. The new generations of membranes are more efficient,” says Anne Le Guennec, CEO of Veolia Water Technologies. “Often developed to meet the demands of industries, which are highly demanding in terms of performance and reducing their impact on the environment, these technologies are also being deployed for municipal use.”
However, it is not only a matter of technology; individuals must make the best use of available treatments by taking the objectives into account as well as local circumstances. This is particularly true for nitrogen treatment. “We are committed to fulfilling our mission,” says Pierre Ribaute, CEO of Veolia's Water Business in France. “The reduction of nitrogen in water below regulatory thresholds is a priority for us to protect the environment. The available technologies allow us to achieve this. However, we must avoid excessive treatment that may lead to over-quality, because nitrogen treatment produces nitrous oxide, a greenhouse gas much more potent than CO2. Lean management, which empowers field teams, should help us achieve a balance.” This balance is crucial for ensuring human health and preserving the planet.
Facing Covid: Innovations For Detection and Prediction of the Epidemic
The global lockdown experienced in 2020 highlighted the crucial role played by Veolia's essential services. The true value of clean drinking water, sanitation in cities, and energy supply were recognized. Without the mobilization of Veolia's teams, both in the field and remotely, additional crises would have compounded the health crisis.
Since then, Veolia's research teams have been devoted to developing analyses to detect and track the epidemic by analyzing wastewater. Their surveillance system, Vigie-Covid-19, has provided valuable insights and supported local decision-making by detecting the virus, monitoring its evolution, and serving as an early warning indicator for its probable circulation in the population.
This system places the analysis results in context by considering factors such as rainfall and population equivalents and comparing them with public epidemiological data.
The temporal evolution of the results helps identify possible resurgences of the epidemic. Vigie-Covid-19, according to Philippe Sébérac, Director of Technological and Scientific Expertise at Veolia, has proven to be “an excellent complement to clinical trials in the fight against the spread of the epidemic, providing interpretable information and dynamics consistent with the incidence rates reported by health authorities in Europe.”
Monitoring wastewater is a promising approach to anticipating viral-origin epidemics. As early as 2003, the World Health Organization recommended this method for polio prevention. The international scientific community now agrees that wastewater “partially reflects the population's health status.”
To relive the year 2020 alongside the Veolia teams, watch the documentary film En premières lignes ("On the Front Lines"), made during lockdown, all over the world:
In Chile, the Risks of Water Pollution Are Acute
Mining activities, industrial waste disposal, and inadequate wastewater treatment have long polluted rivers and groundwater, making them unfit for human consumption and damaging fragile ecosystems. Agricultural practices such as the use of pesticides and fertilizers also contribute to water pollution, posing risks to human and wildlife health.
To address these challenges, Aguas Andinas, a subsidiary of Veolia in Chile, has embarked on the construction of a comprehensive water service, particularly in and around Santiago, ranging from drinking water supply to wastewater treatment, effectively putting an end to the waterborne disease outbreaks that were once frequent there.
Aguas Andinas has made significant contributions to the sanitation of the Mapocho River. Until 1999, only 3 percent of Santiago's wastewater was treated, with the rest being discharged into the river, resulting in devastating consequences for both the ecosystem and public health. Thanks to initiatives such as the Mapocho Urbano Limpio project, which eliminated wastewater discharges into the region's main watercourses, the situation has radically changed in just over a decade.
The benefits are tangible, with a rapid decrease in epidemics being the most prominent. A study by the University of Chile revealed that mortality due to diarrheal diseases among preschool children dropped from 3.8 per 100,000 inhabitants in 1990 to 0.6 in 2003, demonstrating the positive impact of sanitation on public health.
Sanitation has also led to improvements in the river's condition and ecosystem. Moreover, the treated water can now be reused for agricultural irrigation, parks, sports facilities, and even for recharging aquifers, thereby enhancing the water resources available for the region.
Consequently, Aguas Andinas has helped to end decades of water rationing. This is a noteworthy benefit amid the new tests posed by climate change, which is reviving the old challenge of an uncertain water supply in the Chilean capital.
Water shortages are a prominent issue throughout the country. The resource is particularly scarce in regions with arid and semi-arid climates. Precipitation is limited and irregular and, combined with its heavy use for economic activities, results in insufficient water resources. Santiago, with 70 percent of its water supply dependent on the Maipo River into which the Mapocho flows, is no exception.
Aguas Andinas has invested in diversifying water sources, tapping into new wells, and storing drinking water. As a result, neighborhoods dependent on the Maipo River have seen their autonomy increase from four hours in 2011 to twenty-four hours ten years later.
These advancements prevented water rationing in 2021, when exceptional heavy rains upstream of the Maipo River caused landslides that heavily contaminated the water, causing problems for its potabilization. Seven million people who escaped water scarcity then realized the impact of the group on their daily lives.
The current objective is to achieve forty-eight hours of neighborhood autonomy and continue all the actions taken to reduce the vulnerability of Santiago.
To avoid a return to strict rationing after more than a decade of drought, Chilean authorities planned adaptation and water conservation measures in 2022, to which Aguas Andinas has contributed.
- Deaton, Angus. The Great Escape: Health, Wealth, and the Origins of Inequality, United Kingdom: Princeton University Press, 2013.
↩︎ - GIONO, Jean. The Horseman on the Roof. New York: Alfred A. Knopf, 1954. ↩︎
- CORBIN, Alain. The Foul and the Fragrant: Odor and the French Social Imagination. Leamington Spa: Berg, 1986. ↩︎
- DE GMELINE, Patrick. Compagnie Générale des Eaux : 1853-1959, De Napoléon III à la Ve République. Paris: Ed. de Venise, 2006. ↩︎
- DEATON, Angus. “La Grande Évasion. Santé, richesse et origine des inégalités”. Idées économiques et sociales, no. 187 (March 2017). ↩︎
- CORBIN, Alain. The Foul and the Fragrant: Odor and the French Social Imagination. Leamington Spa: Berg, 1986. ↩︎
- DEATON, Angus. “La Grande Évasion. Santé, richesse et origine des inégalités”. Idées économiques et sociales, no. 187 (March 2017). ↩︎
- DE GMELINE Patrick. Compagnie Générale des Eaux : 1853-1959, De Napoléon III à la Ve République. Paris : Ed. de Venise, 2006. ↩︎
- Ibid. ↩︎
- Ibid. ↩︎
- Ibid. ↩︎
- Ibid. ↩︎
- Ibid. ↩︎